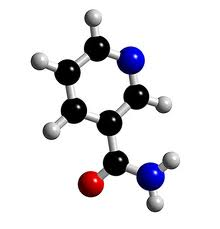
Niacin (NYE-uh-sin) is a B vitamin (vitamin B3) that is essential to cell metabolism. It occurs in two forms, nicotinic acid and nicotinamide, also called niacinamide. The only structural difference between the two compounds is that a hydroxyl group (-OH) in nicotinic acid is replaced by an amino group (-NH2) group in nicotinamide. Lack of niacin causes a disease called pellagra. Pellagra was common throughout human history among poor people whose diet consisted almost entirely of corn products. Those corn products did not supply adequate amounts of niacin, causing symptoms such as diarrhea, scaly skin sores, inflamed mucous membranes, weakness, irritability, and mental delusions. In some cases, people with niacin deficiency develop reddish sores and rashes on their faces. Mental hospitals were full of people who seemed crazy, but who were actually suffering from a dietary deficiency. Thousands of people died from pellagra every year. Nicotinic acid was first isolated by the Polish-American biochemist Casimir Funk (1884–1967) in 1912. At the time,
Funk was attempting to find a cure for another dietary disease known as beriberi. Since nicotinic acid had no effect on beriberi, he abandoned his work with that compound. It was left, then, to the Austrian-American physician Joseph Goldeberger (1874–1929) to find the connection between nicotinic acid and deficiency diseases. In 1915, Goldberger conducted a series of experiments with prisoners in a Mississippi jail and found that he could produce pellagra by altering their diets. He concluded that the disease was caused by the absence of some factor, which he called the P-P (for pellagra-preventative) factor. The chemical structure of that factor was then discovered in 1937 by the American biochemist Conrad Arnold Elvehjem (1901–1962), who cured the disease in dogs by treating them with nicotinic acid.
Niacin is synthesized naturally in the human body beginning with the amino acid tryptophan. Tryptophan occurs naturally in a number of foods, including dairy products, beef, poultry, barley, brown rice, fish, soybeans, and peanuts. People whose diet consists mainly of corn products do not ingest adequate amounts of tryptophan, so their bodies are unable to make the niacin they need to avoid developing pellagra. It takes about 60 milligrams of tryptophan to produce 1 mg of niacin.
Niacin plays a number of essential roles in the body. It is necessary for cell respiration; metabolism of proteins, fats, and carbohydrates; the release of energy from foods; the secretion of digestive enzymes; the synthesis of sex hormones; and the proper functioning of the nervous system. It is also involved in the production of serotonin, an essential neurotransmitter in the brain. Niacin deficiency disorders occur as the result of an inadequate diet, consuming too much alcohol, and among people with certain types of cancer and kidney diseases. Physicians treat niacin deficiency diseases by prescribing supplements of 300 to 1,000 milligrams per day of the vitamin. Overdoses of niacin can cause a variety of symptoms, including itching, burning, flushing, and tingling of the skin.
The Benefit of Vitamin B3
· Required for energy metabolism, enzyme reactions, skin and nerve health, and digestion.
· High doses of nicotinic acid (3 g daily) can lower cholesterol (reduce LDL and triglycerides and increase HDL) and reduce the risk of heart attack and stroke; high dosages should be supervised by a physician.
· Defi ciency causes pellagra, the symptoms of which are skin rash, diarrhea, dementia, and death.
· Defi ciency may be caused by poor diet, malabsorption diseases, dialysis, and HIV.
· Drugs that deplete vitamin B3: antibiotics, isoniazid, and 5-Fluorouracil (chemotherapy).
· High-dose niacin, taken along with statin drugs (i.e., lovastatin), may increase the risk of rhabdomyolysis (muscle degeneration and kidney disease).
· Most people get adequate niacin from diet and/or a multivitamin; supplements may be recommended for those with high cholesterol.